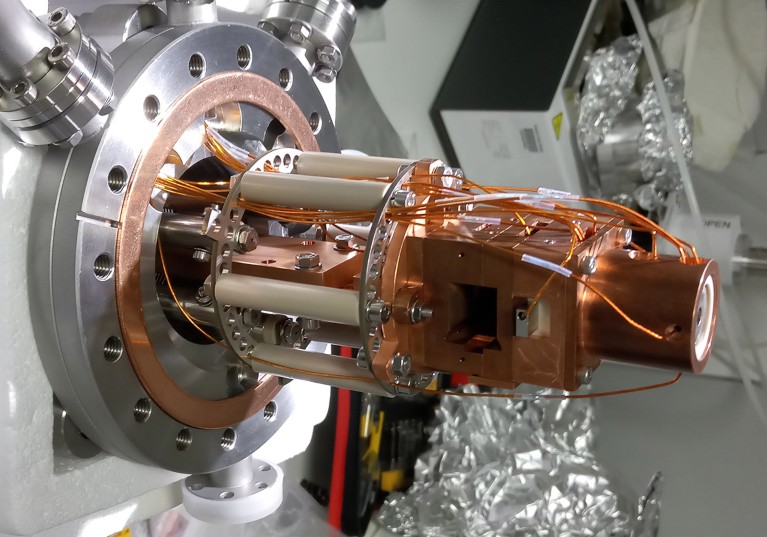
In his lecture after winning a share of the 2002 Nobel Prize in Chemistry, John Fenn described his work as creating “wings for molecular elephants”.
Fenn pioneered the use of a method called electrospray ionization (ESI) to make intact proteins — among nature’s beefiest biomolecules — literally fly, transferring them from complex mixtures into gases and then into mass spectrometers for extensive analysis. Alongside the research of co-recipient Koichi Tanaka, Fenn’s work1 made it possible for scientists to dive deep into the chemical composition — and therefore the sequences, chemical modifications and molecular partners — of whole proteins, using mass spectrometry.
Such data can be invaluable for basic research and biopharmaceutical development — but not protein-structure determination. A growing number of researchers, however, are enthusiastic about the idea of hooking up the technique to a technology that can fill that gap. Using ESI mass spectrometry as an ‘air traffic control system’ to facilitate the take-off, flight and gentle touchdown of intact proteins, in preparation for state-of-the-art methods such as cryo-electron microscopy (cryo-EM), could greatly expand the range of protein structures that can be solved with these powerful but finicky methods. Yet whether it’s possible to land Fenn’s winged molecular elephants safely has remained unclear.
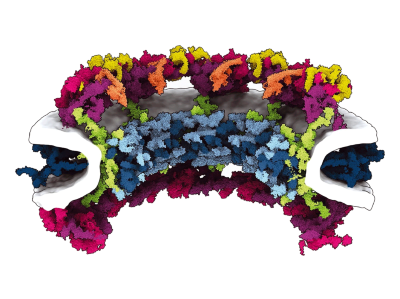
Catching proteins at play: the method revealing the cell’s inner mysteries
Much excitement, therefore, accompanied an August preprint2 from researchers led by physical chemist Stephan Rauschenbach at the University of Oxford, UK. It presented a near-atomic-resolution cryo-EM structure for the enzyme β-galactosidase after preparation with a mass-spectrometry-based approach known as electrospray ion-beam deposition (ES-IBD). The sugar-metabolizing enzyme is one of the best-characterized proteins, making it an ideal test bed for whether ‘soft landing’ mass-spectrometry methods such as ES-IBD can deliver the goods. By tuning the acceleration of a protein as it travels through the mass spectrometer, soft-landing methods aim to limit the force with which the protein arrives at its final destination, thereby minimizing the resulting damage. “Everybody can get a good structure of β-galactosidase — but not after taking it through a mass spectrometer, landing it and visualizing it,” says chemist Carol Robinson, who collaborated with Rauschenbach and is also at the University of Oxford.
The researchers’ results revealed a protein that was somewhat crumpled and dehydrated, but that still closely resembled conventional cryo-EM structures. A July preprint3 from a team led by biomolecular chemist Joshua Coon and structural biologist Timothy Grant, both at the University of Wisconsin–Madison, also reported natural-looking — albeit moderate-resolution — cryo-EM structures for multiple proteins.
Enthusiasts see the possibility of a facile sample-preparation method that allows researchers to generate near-atomic-resolution protein structures with unprecedented precision and efficiency. “It has the potential to be the default way people prepare samples for cryo-EM,” says Coon. Other modes of structural analysis could also benefit, including single-molecule methods that actively monitor the dynamics of flexible proteins. But few groups have made headway with soft-landing mass spectrometry, and the promising results that have been obtained are insufficient to allay concerns that proteins reaching the microscope do not fully retain their natural structure. “It’s a very exciting subfield,” concludes Alexis Rohou, a structural biologist at the biotechnology firm Genentech in South San Francisco, California. “But there are many, many things yet to be overcome.”
A team effort
The marriage of ESI mass spectrometry and cryo-EM is the product of difficulties in two fields.
‘Native’ protein analysis with ESI mass spectrometry entails ejecting proteins from a liquid environment to form airborne ‘gas phase’ particles in a vacuum. This allows researchers to study the biochemical characteristics of intact proteins, as opposed to smaller chunks called peptides, but whether protein structures are fundamentally disrupted by this transition has been the subject of a long-standing debate.
“People were saying to me, ‘You can’t really believe that this looks anything like it does in crystallography or in electron microscopy — surely being in the gas phase has ruined the structure to some extent,’” says Robinson, a specialist in native mass spectrometry. She was convinced otherwise, however, and early experiments supported her view. In 2003, for instance, chemist R. Graham Cooks and his colleagues at Purdue University in West Lafayette, Indiana, generated arrays of soft-landed enzymes that remained functional despite their arduous journey4. Around a decade later, Robinson’s team used transmission electron microscopy (TEM) to show that the structural features of well-studied protein complexes were generally preserved after soft-landing mass spectometry5.
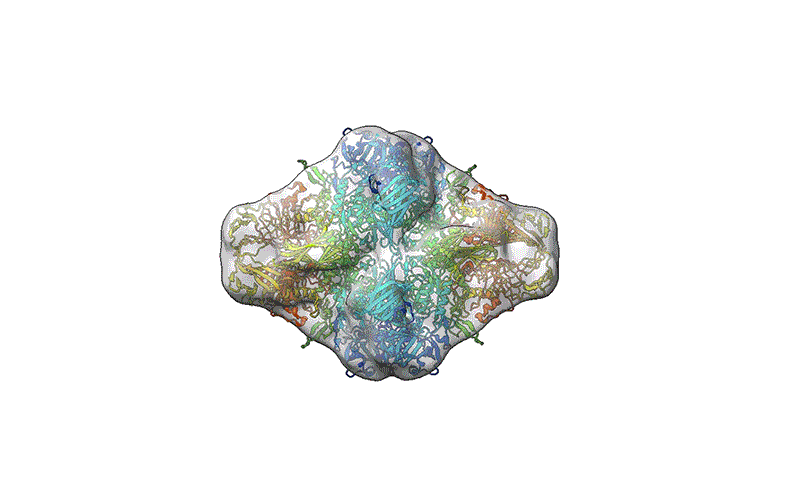
TEM is not suitable for defining the structure of protein molecules at high resolution, but cryo-EM is. In cryo-EM, large numbers of protein molecules are trapped in a thin layer of glass-like ice on a sample grid under conditions that preserve their fine structural features. These frozen protein molecules are imaged at different angles, and then the images are computationally reconstructed into a 3D shape. A good cryo-EM experiment can reveal protein structures with atomic resolution, and the method is now a mainstay of structural biology, with more than 15,000 structures deposited in the world’s repository for protein structures: the Protein Data Bank.
But cryo-EM users have a struggle of their own: sample preparation. “At least half the time, you just can’t get it to work,” says Grant. “And for certain proteins, it’s all the time.” At the freezing stage, protein specimens exist in a thin film of solution that leaves them exposed to air, which can induce protein unfolding and degradation, Grant says. This air–water interface can also cause proteins to preferentially adopt specific orientations. Without a diversity of orientations, it becomes impossible to generate a high-quality cryo-EM reconstruction. Soft-landing mass spectrometry could help to eliminate that bias.
Furthermore, by including soft-landing mass spectrometry in the earliest stages of sample preparation, cryo-EM users could spare themselves the trouble of purifying their proteins and instead pluck them directly from samples on the basis of the protein’s size and biochemical characteristics. “Maybe you could amplify a single population and only deposit that on a grid, or only deposit that in one region of the grid so that another region has proteins in a different state,” says biochemist James Evans, who is part of the leadership team for the Pacific Northwest Cryo-EM Center in Portland, Oregon.
The successful integration of soft-landing mass spectrometry with cryo-EM could therefore resolve two pressing issues — the gas-phase controversy and protein-sample preparation — at a stroke. But getting to that point has proved harder than expected.
Taking the leap
With any aerial routine, one of the biggest challenges is to make a perfect landing — and so it was with soft-landing mass spectrometry. “We started more than 20 years ago,” recalls Klaus Kern, a chemist at the Max Planck Institute for Solid State Research in Stuttgart, Germany, who supervised Rauschenbach’s initial work on ES-IBD as a postdoc. “It took 10–12 years before it really started working”.
The instrumentation itself can be built around a commercial mass spectrometer — both Coon and Rauschenbach have used Orbitrap instruments, from US biotechnology company Thermo Fisher Scientific, as a foundation. But considerable tuning and modification are required to protect the integrity of the protein molecule during transit and to manage its speed and eventual impact with the sample grid. Careful optimization of both the sample-preparation conditions and the surface of the ‘landing pad’ are also required.
Coon recalls reaching out to Grant early in their collaboration to show off some of the data his team had produced. “We were all proud of these images, and we said, ‘Tim, what do you think?’ And he’s like, ‘Your proteins are shit, they look kind of like you threw a tomato at a wall’,” Coon says. His team spent about 18 months testing different instrumentation and sample and surface conditions before finding a formula that worked: coating sample grids with an ultra-thin layer of glycerol to capture the landed proteins. Using TEM, the researchers confirmed successful deposition of seemingly intact GroEL — a cylindrical chaperone protein that enables the folding of other proteins — with modest resolution. But the workflow was incompatible with cryo-EM, because glycerol produces too much noise in the images, and so they went back to the drawing board to make their process more cryo-friendly. The results were published in April 20226.
Months later, Rauschenbach and his colleagues described an approach that came closer to a standard cryo-EM workflow7. They deposited gas-phase proteins onto a room-temperature, unmodified grid, which they then plunged into liquid nitrogen to freeze the proteins in place without forming an ice layer. Rauschenbach was pleased to note that the structure of β-galactosidase looked more or less correct, and his team saw evidence of features such as α-helices and β-sheets. “Even at room temperature, something was retained,” he says. “But the resolution was not good enough to fit models.”
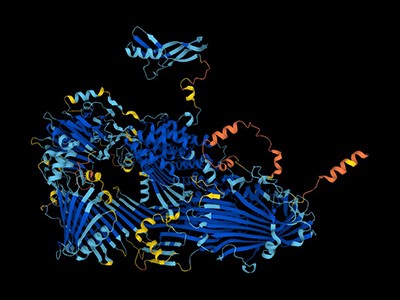
‘The entire protein universe’: AI predicts shape of nearly every known protein
Rauschenbach and Coon independently realized that freezing the proteins as soon as they leave the vacuum environment of the mass spectrometer could solve that problem. Both teams described important progress towards the use of soft-landing mass spectrometry for cryo-EM sample preparation in the July and August preprints2,3. Coon and Grant’s group achieved3 this by landing the proteins on a grid that it had pre-chilled to 190 °C. The researchers then restored the grid to atmospheric pressure before plunging it into liquid nitrogen. By contrast, Rauschenbach’s team coated its mass-spectrometry-deposited proteins with a thin ice layer by introducing low levels of water vapour into the sample chamber, which quickly froze on the surface of the pre-chilled grid2. Rauschenbach says that his team’s ice-free samples tend to form problematic artefacts, “but when you embed them in ice, you get the structure”.
Damage control
The results have led to cautious optimism. Both groups saw considerable improvements in the resolution that they could obtain for β-galactosidase, and Coon’s group also obtained a higher-quality 3D structure for GroEL compared with the earlier work using glycerol. In fact, Rauschenbach and his colleagues achieved a resolution of 2.6 ångströms — about the length of a hydrogen bond, and slightly poorer than results obtained with conventional cryo-EM samples.
But his team’s reconstruction of β-galactosidase was somewhat compacted relative to the protein’s known structure. The researchers surmised that the enzyme’s journey through a harsh vacuum environment stripped away the water molecules that surround proteins in nature, causing it to dehydrate and shrivel. “The important point is [that] this is not the solution structure — it is a gas-phase protein landing on a cold surface,” Rauschenbach explains. In their preprint, the researchers showed that they could largely restore the ‘correct’ structure using an algorithm that simulates protein hydration.
The significance of this dehydration for sample preparation is unclear. On the one hand, these results largely vindicate researchers such as Robinson, who posited that gas-phase proteins generally retain their structure. On the other hand, structural biologists seeking a route for preparing intact native proteins are still awaiting more evidence. “How much dehydration damages the protein is, I think, a somewhat open question,” says Grant. He and his team are continuing to test other proteins in their workflow — Coon says that they prepare four to five new grids daily — in the hope that they can improve understanding of what happens during the soft-landing mass-spectrometry process.
But Tanmay Bharat, a structural biologist at the MRC Laboratory of Molecular Biology in Cambridge, UK, who collaborated with Rauschenbach, is optimistic about the method already. “It’s a very good starting point for improving the process even more,” he says, although he notes that further work will be required to turn it into a robust and generalizable protocol for protein cryo-EM. Both teams are looking into opportunities to use mass spectrometry with proteins that retain at least a partial water coating and can therefore be frozen in a more natural state.
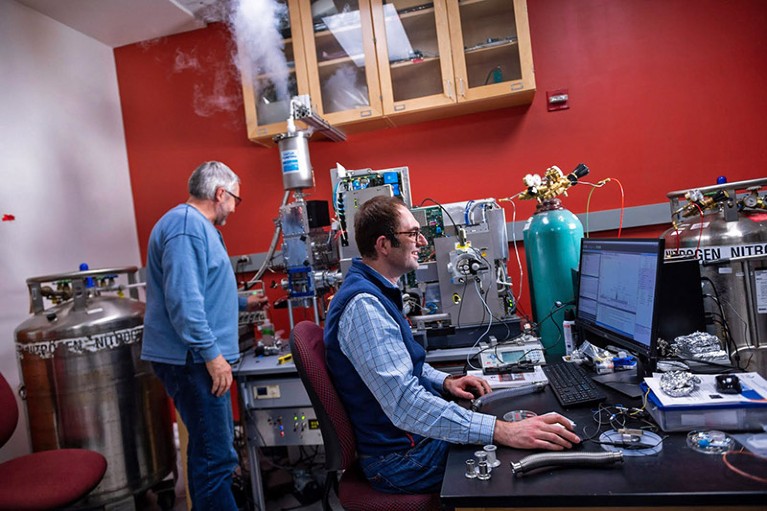
Other groups have begun testing the waters of soft-landing mass-spectrometry. For example, Rohou and his colleagues at Genentech are working with an ‘ion mobility’ deposition method developed by life-science company IonDx in Monterey, California, which allows the sorting of proteins that remain fully hydrated and therefore could retain more native structures. The team still struggles to land intact proteins on its grids, says Rohou, but “they have frozen water and protein in them, and we can recognize proteins in each individual droplet.”
Similarly, Evans and his collaborator Ljiljana Paša-Tolić, a mass-spectroscopy specialist at Pacific Northwest National Laboratory in Richland, Washington, who have also stumbled with soft-landing mass spectrometry, are exploring an alternative approach. Known as Structures for Lossless Ion Manipulation (SLIM), it operates under ‘softer’ vacuum conditions and could therefore reduce the loss of water. “You’re still under some vacuum, but … you may be able to create and keep a shell of hydration or a salt shell even around the protein,” says Evans.
A wide-open runway
For cryo-EM enthusiasts, the current state of limbo is both exciting and frustrating. “It’s almost like it’s binary — you can either do it all or do nothing,” says Grant. “And right now, nobody’s done it all.” And the consequences of turning this into a robust, lab-ready technique could be huge.
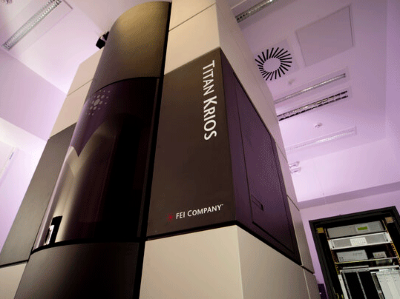
The must-have multimillion-dollar microscopy machine
Integrated into the cryo-EM workflow, soft-landing mass spectrometry could allow more-elaborate experiments. “You could lyse a cell and basically pick out complexes of certain molecules with certain other molecules,” says Bharat, or more precisely characterize the interactions between drug candidates and target proteins. The integration could also make small proteins more amenable to cryo-EM analysis. Such proteins are typically invisible in the relatively thick layers of ice formed by current plunge-freezing methods, Rohou explains. A mass-spectrometry-based method that either eliminates the need for ice or reduces it to a thin shell around the protein could make these proteins tractable for high-resolution analysis.
But soft-landing mass spectrometry is already creating exciting possibilities for protein analysis at the single-molecule level. Kern, Rauschenbach and their colleagues initially began exploring soft-landing mass spectrometry as a preparative tool for characterizing proteins and other biomolecules with an approach called scanning tunnelling microscopy (STM). This involves a tiny, ultrasharp probe being manoeuvred over an immobilized sample while a voltage is applied; bumps and divots in the sample surface produce changes in the resulting current, which can then be mapped to determine the underlying sample structure. In 2020, Kern and his colleagues demonstrated for the first time that STM could reveal the structure of complex carbohydrates that had been deposited by soft-landing mass spectrometry8. His team is now extending the approach to analyse glycoproteins in unprecedented detail. “We can directly see what glycan is attached to what amino acid in a polypeptide,” says Kern.
Kern’s team is also integrating soft-landing mass spectrometry with a relatively obscure STM variant known as low-energy electron holography (LEEH), to recover information about flexible proteins that can adopt multiple conformations. In LEEH, the ultrasharp probe serves as an electron source that bombards a target molecule on an ultraclean layer of graphene, producing an interference pattern that can be reconstructed to determine the target’s 3D structure. Theoretically, the process can achieve near-atomic resolution, Kern notes. But his team has already clearly distinguished different structural configurations of a protein of interest — a scenario that would create only blurry images in cryo-EM9.
These developments are just the beginning for soft-landing mass spectrometry, and for Rauschenbach, that’s the most exciting aspect: the untapped versatility. “You can do all types of chemistry, deposition and analysis methods,” he says. “We can use it for so many things.”
"soft" - Google News
October 16, 2023 at 06:31PM
https://ift.tt/fi13Iem
Soft-landing methods aim to simplify structural biology - Nature.com
"soft" - Google News
https://ift.tt/25xzehw
https://ift.tt/mj4K0yS
Bagikan Berita Ini
0 Response to "Soft-landing methods aim to simplify structural biology - Nature.com"
Post a Comment